Department Spin and Topology in Quantum Materials
Spin and Topology in Quantum Materials
We explore the fundamental principles, concepts, and materials underpinning future information technologies. In contrast to conventional materials such as silicon or permalloy, quantum materials exhibit an intrinsic entanglement of charge, spin, orbital, and lattice degrees of freedom. This interplay gives rise to emergent phenomena including topological protection, strong electron correlations, and superconductivity.
Topological protection emerges from nontrivial topology in the electronic band structure, leading to robust surface states that are less susceptible to scattering. These surface states feature a distinctive spin texture in momentum space, characterized by spin-momentum locking. In real space, topologically nontrivial magnetic textures—such as skyrmions—can form in certain magnetic materials, opening further possibilities for functionality in transport phenomena.
These quantum phenomena are key enablers for next-generation technologies ranging from spintronics to topological quantum computing. We investigate them across multiple length, time, energy, and temperature scales, and under high magnetic fields. Our experimental approach focuses on techniques sensitive to electron spin, including spin- and angle-resolved photoemission spectroscopy (ARPES), photoemission electron microscopy (PEEM) combined with x-ray magnetic dichroism, as well as soft x-ray magnetic scattering and absorption in vector magnetic fields.
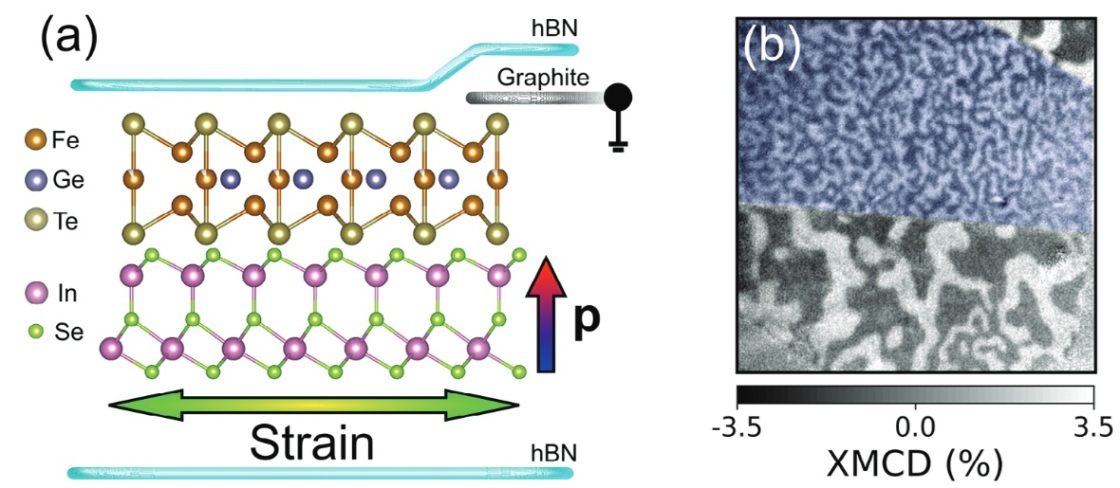
Van der Waals heterostructures allow atomically thin layers to be stacked with relaxed epitaxy, but strain-free integration is not always achieved. In a collaboration with Oxford university, exfoliated piezoelectric α-In₂Se₃ is used to strain the van der Waals ferromagnet Fe₃GeTe₂, leading to higher domain wall density, reduced transition temperatures (by 5–20 K), and increased coercivity. Structural changes are supported by Raman analysis of α-In₂Se₃/graphite heterostructures. DFT and spin dynamics simulations indicate a 0.4% compressive strain in Fe₃GeTe₂, reducing its exchange stiffness and anisotropy. To the right, an XPEEM image of the magnetic domain is seen where the blue part marks the heterostructure. This approach suggests a route to strain-engineer 2D interfaces using piezoelectric layers.
R. Fujita et al., Adv. Funct. Mater. 34, 2400552 (2024).